Mixed doubles victory revives China's golden sweep hopes in table tennis
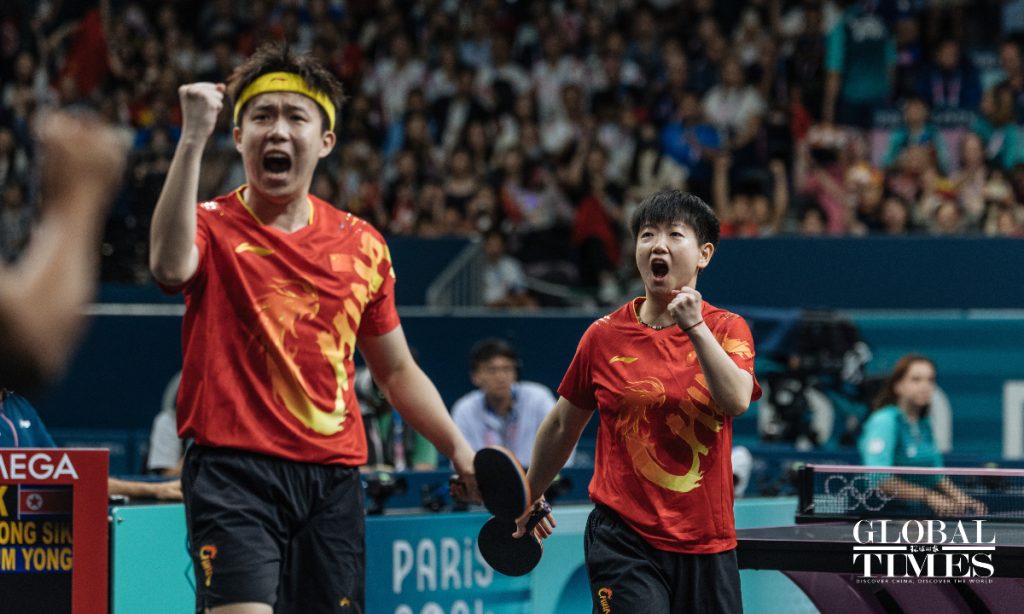
Chinese table tennis stars Wang Chuqin and Sun Yingsha captured the mixed doubles gold medal on Tuesday, defeating North Korea's Ri Jong-sik and Kim Kum-yong 4-2 in a thrilling final.
This victory marks the sixth gold medal for China at the Paris 2024 Olympics and restores the pride of the Chinese table tennis team, known as the Dream Team.
The Chinese team was one mixed doubles gold medal short of a clean sweep at the Tokyo Olympics. Wang and Sun's win sets the stage for a possible golden sweep in Paris.
The much-anticipated event attracted several dignitaries, including International Olympic Committee President Thomas Bach.
Ahead of the Paris Olympics, Sun emphasized the importance of the mixed doubles gold, stating it was even more significant than the singles events.
To prepare, Sun sought help from Ni Xialian, a 61-year-old former Chinese player now representing Luxembourg. Ni's playing style is similar to Kim's, making her an ideal training partner.
The North Korean pair had rarely competed in international tournaments until the Paris Olympics.
As the lowest-ranked 16th seed among the mixed doubles pairs, Ri and Kim's journey to the final was remarkable.
They earned their Paris spot through the Olympic qualifiers and were dubbed the "mysterious team" due to their limited exposure in international competitions.
The North Korean pair's surprise elimination of Japan's second-seeded duo, Tomokazu Harimoto and Hina Hayata, in the first round exemplified their unpredictable nature.
An unexpected incident after the final means Wang has to pick up a new paddle in the singles matches, after overenthusiastic photographers broke his winning racket when capturing the duo's celebrations.
"The photographer broke the racket... such behavior probably shouldn't have happened," Wang told reporters.
"I felt a bit out of control in that situation and couldn't understand why it happened. But it did, and there's nothing we can do about it... and I can still play with my spare racket."
Among the five table tennis disciplines, the mixed doubles has been the most unpredictable for Team China in terms of results.
At the Tokyo Olympics, China missed out on the mixed doubles gold when Xu Xin and Liu Shiwen were defeated by Japan's Jun Mizutani and Mima Ito, leaving the Chinese team one gold short of a complete sweep.
"Securing the mixed doubles gold has not only started the table tennis team's Olympic campaign on a high note, but also relieved the immense pressure on the athletes and coaches," Mao Jiale, a Chengdu-based sports commentator, told the Global Times.
"With the mixed doubles title in hand, the national team can now approach the upcoming singles and doubles events with solid confidence and focus on achieving their goal of winning five gold medals in Paris."